A program to rebuild America’s infrastructure could be realized within the next year. That means more and more bridge designers, engineers, and contractors could be asked to retrofit (and build) bridges to make them earthquake proof.
Here’s everything you need to know about earthquakes, how they impact bridges, and the latest things being done to make bridges and the utility infrastructure they support safer (and in some cases, indestructible) during earthquakes.
Earthquakes by the numbers
How likely is it that your area will be affected by an earthquake? Check out these statistics about seismic activity in the United States:
- According to data from the United States Geological Survey, there have been, on average, 18 major earthquakes of magnitude 7.0-7.9 and a substantial one of magnitude 8.0 or greater per year since 1900 in the U.S.
- The same source reports that the overall average annual number of earthquakes has remained relatively stable. However, activity in some states has increased dramatically in the last few years.
- Western states are more affected by earthquakes than those in other parts of the country. The top 10 in 2015 were Alaska (1,575 quakes of magnitude three or greater), Oklahoma (888), Wyoming (198), Nevada (172), California (130), Kansas (60), Hawaii (53), Idaho (38), Texas (21), and Montana (19).
- Only 10 states had no earthquakes during that period. Most are on the east coast and upper mid-west.
- While most states have had consistent seismic activity over the last half-decade, some have experienced a significant uptick: Oklahoma (41 in 2000 to 888 in 2015), Wyoming (43 to 198), Nevada (38 to 172), Kansas (zero to 60), Idaho (seven to 38), Montana (seven to 19), and Texas (nine to 21).
While there’s no conclusive scientific evidence, some experts believe the localized increase in quakes is the result of human activity, including things like fracking.
Check it out: The United States Geological Survey website offers complete data and information about earthquakes in the United States and across the globe.
Earthquake retrofitting strategies
Much has been learned about earthquakes in the last 20 to 30 years. Significant improvements in construction techniques and building materials have made new structures built during that time much safer. Many of these innovations can be applied to retrofitting older bridges.
The first thing engineers rehabilitating older structures need to consider is what they want their seismic retrofit to accomplish.
In order from least intensive to most, the options include:
- Public safety. In this case, the ultimate goal is to save lives. The structure may end up damaged in a quake, but it does not collapse on or under vehicles or pedestrians. It is critical that steps are taken so the bridge can be safely evacuated after a seismic event. In a worst-case scenario, the bridge may require tear-down and replacement. It is rare for retrofits to aim this low. Most target a minimum level of structural survivability.
- Structural survivability. The goal is that the structure is updated so the public is protected during a quake, can escape safely afterward, and the structure is repairable after the event is over. This is the most common form of retrofit undertaken today.
- Structural functionality. In this step up in the retrofitting scale, the bridge is undamaged and able to be used almost immediately after a seismic event. Only minor repairs should be required.
- Structure is unaffected. This is the ultimate level of retrofit. It is generally reserved for historic bridges and structures that are significant and would be a serious loss to a community if destroyed.
A few strategies that can be applied to existing bridges so they’re more likely to withstand a quake:
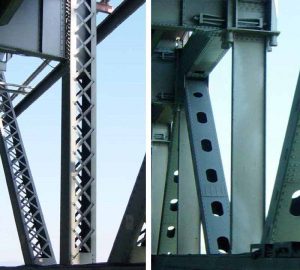
San Francisco-Oakland Bay Bridge retrofit replacing riveted lattice elements with bolted steel plates.
- Strengthening the structure using cross braces and other reinforcements. A few bridges can benefit from strengthening certain sections, although for most older structures, a bigger factor is making them more flexible.
- Reducing the impact of seismic activity by using damping or structural isolation systems. This prevents rigid structural components from banging into each other, which is a leading cause of earthquake damage.
- Increasing the flexibility by retrofitting sections to bend or twist if doing so will help the bridge survive a quake. These sections can be replaced after seismic activity is over to return the bridge to structural integrity.
- Weakening certain sections of the structure in order to preserve the rest. Sometimes it’s impossible to retrofit a bridge so the whole thing can survive a quake. In those cases, it makes sense to weaken areas so they crumble and break, leaving the rest of the bridge standing. Repairing these sections can happen more quickly after a quake than rebuilding a whole bridge.
- Adding sliding elements that allow different sections of the structure to move independently so they don’t crack, break, or bang into each other.
- Incorporating dampers that can absorb some of the forces that result from seismic activity.
Photo above by user: Optigan13 Based on en:User:Leonard G. [CC SA 1.0], via Wikimedia Commons.
A few common bridge retrofitting techniques:
- External post-tensioning. This technique has been tested and used on new non-bridge structures for about 10 years. Unbonded post-tensioning high-strength steel helps increase the capacity of structural elements such as beams, columns, and the joints that connect them. This technique has been recently tested on bridges in California.
- Base isolators. These structural elements separate the structure from the shaking ground. Think of it as a kind of vibration control. In a retrofit, material is removed from the foundation and replaced by isolating pads. Care must be taken to ensure that the isolated elements sway relative to each other in a safe way.
- Supplementary dampers. These dampers transform motion energy into heat. This helps dissipate the effect shaking can have on a bridge. Think of them as like shock absorbers in a car.
- Additional structural support, reinforcements, and enhancements. Some older bridges benefit from reinforcements that strengthen them. Others are more likely to make it through a seismic event if rigid elements are replaced by more flexible ones that allow the bridge to sway. One addition every bridge can benefit from is enhanced protection for utilities that are supported by bridges.
- Connectors between sections. One of the best ways to protect the elements on an aging structure are to separate them and protect the two elements from banging into each other using dampers.
Building earthquake-proof bridges
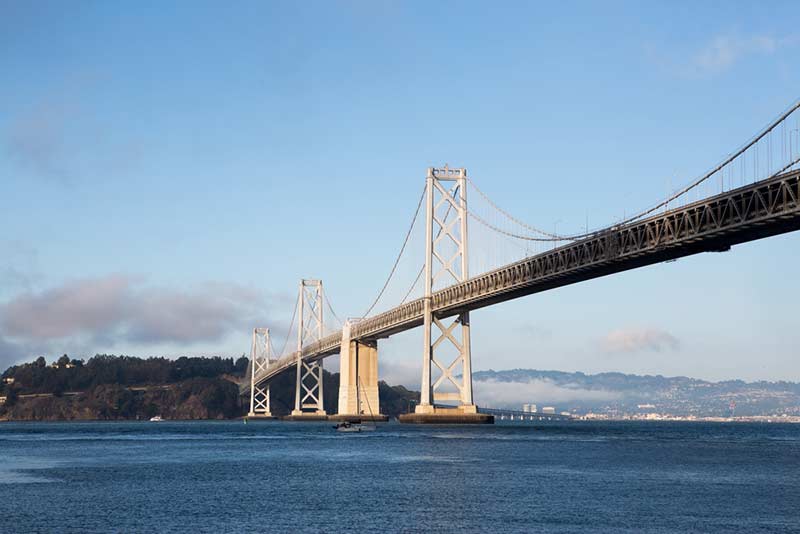
One way to learn more about retrofitting bridges so they’re earthquake proof is to check out the cutting-edge techniques used on two innovative bridges constructed in high-seismic areas.
Let’s start by looking at the replacement for the San Francisco-Oakland Bay bridge that was damaged decades ago in a major quake.
More than a quarter million vehicles cross the span every day. Because of this, engineers were challenged to design a bridge that could be returned to service as quickly as possible after seismic activity. Not only did they plan a bridge that could make it through the major earthquakes that are predicted to happen during the projected 150-year lifespan of the bridge, they also planned for it to withstand the worst possible seismic events over a 1,500-year period.
It is anticipated that traffic can be returned to the bridge within hours of a quake rather than days, weeks, or months, as has been standard in the past.
The bridge is supported by extraordinarily strong yet flexible steel cable bundles that twist wire upon wire into cables. These cables are then bound together in bundles that will maintain flexibility yet stay strong in a seismic event.
The structure has also been strengthened with the addition of steel plates, new types of rivets and bolts, and extra bracing. Engineers also inserted rollers under the roadway along with viscous dampers that help absorb the shock from earthquakes so the bridge can move without doing damage to itself.
The structure has been built in multiple segments. Each is supported on its own pier. The segments meet closely enough to make the road continuous, but they aren’t fastened to one another. Instead, a small gap has been left between them, which lets them move independently during an earthquake. Large rubber bumpers between the sections protect the segments from damaging each other should they come into contact while being shaken.
One of the bridge’s most innovative safety features are hinge pipe beams that connect the segments of the bridge to each other. They are engineered to keep the segments aligned during a seismic event. One section of each pipe is a soft steel fuse. It’s designed to bend under pressure, so the beam can “give” a little during an earthquake. Once a period of seismic activity is over, any deformed fuse sections will be replaced so the hinge pipe beams will be ready for the next episode.
Bridge designers also took steps to protect critical utility infrastructure. A long corridor runs the entire length of the bridge. Special care is being taken to protect the electrical wiring that powers the lights on the bridge, along with other cables and pipelines that serve utility customers.
Did you know? These innovations go well beyond one of the steps workers took when they repaired the original San Francisco Oakland Bay Bridge after a previous earthquake? They welded a troll into the bridge to ensure good luck.
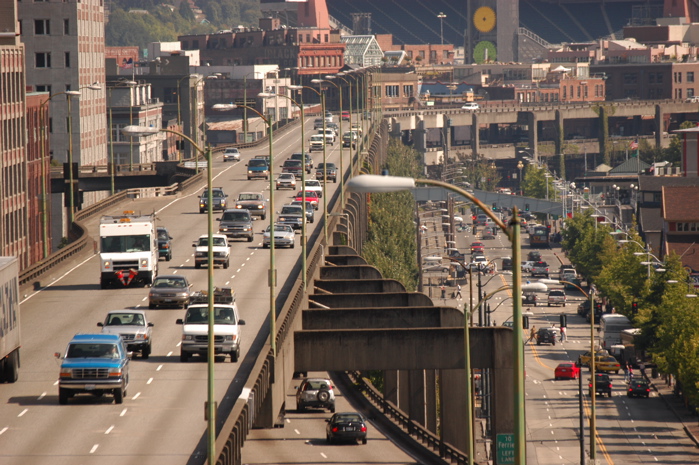
Photo above by user: wac [CC SA 1.0], via Wikimedia Commons.
The Alaskan Way Viaduct in Seattle is another example of a bridge in an earthquake-prone area that uses cutting-edge
engineering techniques to keep it safe. Much like the San Francisco-Oakland Bay Bridge, this one has been designed to make it through a quake with little or no damage, so it can be returned to service quickly after the event is over.
One of the innovative safety features leveraged by this structure are rods made with a flexible nickel-and-titanium alloy inserted into the concrete columns. They automatically return to their original shape after being impacted by outside forces.
The concrete used to house these rods is unique as well. It’s a special composite made with fibers that let it bend without cracking and crumbling.
These innovative materials are 90 times more expensive than typical ones. However, they’re only used in the most vulnerable areas of the bridge, rather than the entire structure. This sensible strategic approach means this “safer” bridge costs less than 10 percent more than a standard one.
Think about it: This minimal added expense is justified because it is significantly less than the price tag of repairing a bridge damaged by a single major quake.
Designers of new bridges being built from the ground up have the freedom to incorporate these novel ideas and others into their structures. For those retrofitting older ones, this may not be possible. Using the newer, more flexible materials and taking steps to isolate sections of bridges from each other could go a long way toward keeping people safe in a quake.
Another big step is to leverage the latest seismic monitoring systems to be able to control traffic on bridges prior to, during, and after a quake. Another relatively easy step: Update the utility infrastructure and protective equipment on bridges to help avoid floods, fires, and electrical outages that could exacerbate earthquake damage.
Finally, bridges in earthquake zones may need to be inspected more often than regulations demand to look for cracks and other signs of seismic damage. Drones and modern lift equipment make virtual and in-person inspections safer, easier, and more cost-effective than ever.